​​​
developing sustainable thermal solutions

Our Research and Initiatives
At Functional Thermal Lab, we are dedicated to advancing fundamental understanding of energy carrier transport through micro/nanostructures and its application for sustainable energy technologies. Our team combines theory and simulation with experiments to solve practical problems.
1. Investigating energy carrier transport under inhomogeneous strain
​
Nanoscale structures can produce extreme strain that enable unprecedented material properties, such as tailored electronic bandgap, elevated superconducting temperature, and enhanced electrocatalytic activity. While uniform strains are known to elicit limited effects on heat flow, the impact of inhomogeneous strains has remained elusive due to the co-existence of interfaces and defects. For this project, our goal is to investigate energy transport under non-uniform strain conditions and establish the underlying relationship between strain gradients and the behavior of various energy carriers, such as phonons, polaritons, and electrons, etc.
2. Exploring abnormal phonon transport phenomenon within ultra-thin nanostructures
​
Fascinating phenomena can occur as charge and/or energy carriers are confined in one dimension. One such example is the divergent thermal conductivity (κ) of one-dimensional lattices, even in the presence of anharmonic interatomic interactions—a direct consequence of the Fermi–Pasta–Ulam–Tsingou paradox proposed in 1955. This length dependence of κ, also known as superdiffusive phonon transport, presents a classical anomaly of continued interest. In this project, through combining nanoscale thermometry with atomic scale lattice vaibrational spectroscopy (such as STEM-EELS), we aim to provide direct experimental evidence of this exotic phonon transport phenomenon.
3. Developing low energy consumption and ultra-sensitive micro/nano sensors
​
The development of low energy consumption and ultra-sensitive micro/nano sensors is essential for enhancing the efficiency, reliability, and sustainability of various technologies and applications across a wide range of industries, from healthcare and environmental monitoring to space exploration and scientific research. These sensors enable precise and real-time data collection while minimizing the energy footprint, making them invaluable in our increasingly connected and data-driven world. To this end, we are currently designing innovative Pirani gas pressure gauge using various strategies.
4. Designing functional thermal metamaterials using additive manufacturing technqiues
​
Thermal metamaterials, including thermal switch, thermal cloaking, thermal shielding devices, have a wide range of applications that can improve energy efficiency, enhance thermal management, and lead to innovative solutions in fields such as electronics, aerospace, healthcare, and environmental sustainability. They have the potential to address critical challenges related to heat control, making them significant in both scientific research and practical applications. To this end, we utilize 3D printing technology for the integrated manufacturing of various types of thermal metamaterials. This simplifies the device manufacturing process, provides excellent mechanical performance, and enables the conformal fabrication of irregular surfaces.
5. Developing ultra-high temperature thermal energy storage technology
​
The lack of affordable and deployable large-scale energy storage is a significant obstacle to supplying on-demand, zero-emission energy for societal decarbonization. High-temperature thermal energy storage stands out as a promising solution due to its low cost and high scalability. However, it faces challenges in meeting all material requirements and system constraints simultaneously, given its inherent complexity. In this project, we are actively seeking the ideal storage material candidate and performing system-level design for this upcoming energy storage technology.
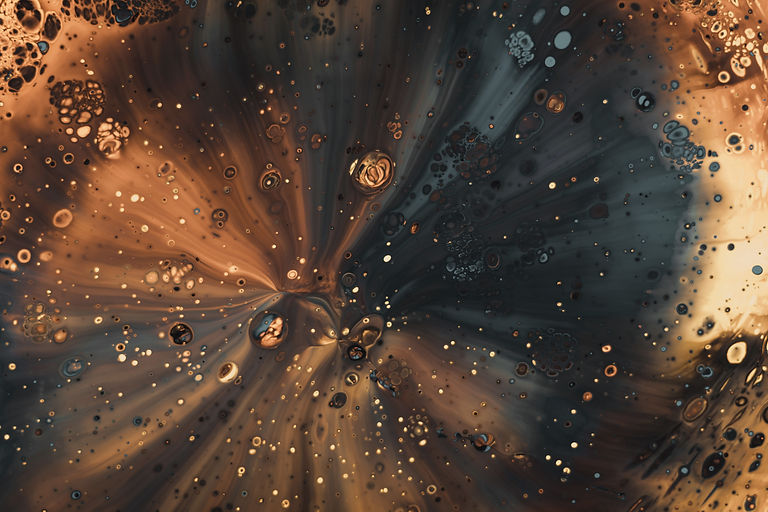